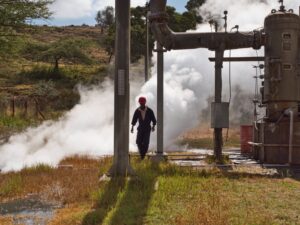
Introduction
Volcanic geothermal resources are powerful candidates for sustainable electricity generation. In 2010, the top ten volcanic geothermal fields provided 50% of the total worldwide installed power generation capacity (Bertani, 2015).
Active continental rift systems are ideal places for geothermal power generation. Steady, rifting-related magmatic processes result in volcanic heat, that is easily accessible near the surface. An example for such an active continental rift is the Main Ethiopian Rift (MER), which is part of the greater East African Rift System (EARS) (Corti, 2009). The EARS is mostly known for its dramatic landscapes with more than 120 active volcanoes (Global Volcanism Program, 2013) such as the Kilimandscharo and the Erta Ale in the Danakil depression with its bubbling lava lake and multi-coloured hydrothermal fields.
With this project we will conduct a geophysical survey in the MER to understand how magma is spatially distributed in the rift, what controls magma transport and storage under magmatic segments and how it influences the formation of geothermal reservoirs. To accomplish this, we will conduct a regional magnetotelluric (MT) survey covering the central MER (Figure 1). The study area encloses the geothermal fields of Tulu Moye, Aluto and Corbetti. These three geothermal fields have been studied by MT in great detail (Gislason et al. 2015, Samrock et al. 2018, 2020a), a pilot power plant is operating at Aluto and geothermal drilling is ongoing at all sites.
Figure 1: Map of the study region in the MER, showing the more than 600 MT measurements from past studies and the layout of the proposed regional survey of this project (red squares). Geothermal prospects are at b) Tulu Moye, c) Aluto and d) Corbetti volcano. Volcanic vents are grouped into vents at the Wonji Fault Belt (WFB) and the Silti Debre Zeyt Fault Zone (SDFZ).
Objectives and previous studies
The new regional-scale MT survey will complement and expand the existing high-resolution MT surveys at Tulu Moye, Aluto and Corbetti. Based on the new data we will compute a multi-scale 3-D electrical conductivity model of the MER that covers depths from the surface of the geothermal fields down to the upper mantle.
The electrical conductivity in the subsurface is predominantly controlled by the presence and composition of fluids and melt and the hydrothermal alteration mineralogy. In volcanic environments MT provides key information about the extent and architecture of magmatic and hydrothermal systems (Samrock et al., 2018): The local models from Aluto and Tulu Moye show both a transcrustal two-level magmatic system with magma ponding in the lower crust, C4, quick rise of magma along weak zones into the shallow crust, C3, and a zone of magma storage and fractional crystallization at 3-5km below the surface, C2 (Figure 2, Samrock et al. 2018, 2020a).
Both models are in striking agreement with petrological findings (Trua et al., 1999; Rooney et al., 2007, Gleeson et al. 2017). Differences in their electrical conductivity reflect different stages of melt evolution and composition (Samrock et al., 2020b). At Aluto and Tulu Moye geothermal wells are drilled through the clay cap, imaged as conductor C1, into the up-flow zone atop of the shallow intrusion C2. However, the big picture that shows the link between continental rifting on a large scale and the formation of hydrothermal reservoirs on a small scale, is still missing.
From the new regional scale 3-D model, we expect to gain new insights into:
-
- how the volcanic systems of Aluto and Tulu Moye are connected to the deeper region of melt generation,
- how magma is spatially distributed beneath the MER,
- what controls magma transport and emplacement under volcanically active sites and
- how it influences the formation of prospective geothermal reservoirs.
The potential of geothermal energy for Ethiopia
Even though volcanoes are often regarded as a hazard, they also provide beneficial resources, such as ore minerals and geothermal energy. Various applications from balneology over greenhouse heating to electricity generation, make geothermal energy beneficial not only for smaller communities, but also for the infrastructure of entire countries. Hence, geothermal energy can be a catalysator for sustainable socio-economic development.
Ethiopia´s geothermal energy resources are estimated to have a power generation potential of about 10’000 MWe and thus bearing the potential to play a key role in Ethiopia’s future. While already nearly 100% of the country´s electricity is generated from renewable energies, 43% of the households still have no access to electricity.
To overcome this energy poverty the Government of Ethiopia launched in 2017 the National Electrification Program, which led to a boost in geothermal energy projects. Contracts were signed to install geothermal power generation facilities with a total capacity of more than 1000 MWe.
If the projects succeed, Ethiopia could become one of the worldwide leading countries in geothermal energy, following in the footsteps of its neighbour Kenya. With an impressive increase of 600 MWe geothermal energy in the past 5 years, Kenya is now number 5 of the top 10 leading countries in geothermal energy worldwide. Today, Kenya can be regarded as an enlightening example for large-scale and successful geothermal development.
Related Publications by the GEG Group
REFEREED PUBLICATIONS IN JOURNALS
PROCEEDINGS REFEREED
References
Corti, G. (2009). Continental rift evolution: from rift initiation to incipient break-up in the Main Ethiopian Rift, East Africa. Earth-Sci. Rev., 96 , 1-53. doi:10.1016/j.earscirev.2009.06.005.
Gíslason, Gestur, et al. “Results of surface exploration in the Corbetti Geothermal Area, Ethiopia.” In World Geothermal Congress (WGC 2015) , Melbourne, Australia. 2015.
Gleeson, M. L., Stock, M. J., Pyle, D. M., Mather, T. A., Hutchison, W., Yirgu, G. and J. Wade, (2017). Constraining magma storage conditions at a restless volcano in the Main Ethiopian Rift using phase equilibria models. J. Volcanol. Geotherm. Res., 337 , 44-61. doi:10.1016/j.jvolgeores.2017.02.026.
Global Volcanism Program, 2013. Volcanoes of the World, v. 4.9.0. Venzke, E. (ed.). Smithsonian Institution. doi:10.5479/si.GVP.VOTW4-2013
Rooney, T., Furman, T., Bastow, I., Ayalew, D. and G. Yirgu (2007). Lithospheric modification during crustal extension in the Main Ethiopian Rift. J. Geophys. Res. Solid Earth, 112. doi:10.1029/2006JB004916.
Samrock, F., Grayver,A.V., Bachmann, O., Karakas, O. and M.O. Saar (2020b). Integrated magnetotelluric and petrological analysis of felsic magma reservoirs: Insights from Ethiopian rift volcanoes. (In Review at EPSL).
Trua, T., Deniel, C. and R. Mazzuoli (1999). Crustal control in the genesis of Plio-Quaternary bimodal magmatism of the Main Ethiopian Rift (MER): geochemical and isotopic (Sr, Nd, Pb) evidence. Chem. Geol., 155, 201-231. doi:10.1016/s0009-2541(98)00174-0